Магнітні нанокомпозити для новітніх технічних та медичних застосувань
За матеріалами доповіді на засіданні Президії НАН України 30 листопада 2022 року
DOI:
https://doi.org/10.15407/visn2023.02.043Ключові слова:
магнітні нанокомпозити, терагерцовий частотний діапазон, антиферомагнітне впорядкування, самоконтрольована магнітна гіпертерміяАнотація
У доповіді розглянуто актуальні тенденції розвитку пристроїв на основі магнітних матеріалів. Підкреслено, що важливим напрямом є розроблення та дослідження новітніх нанокомпозитів, використання яких у зазначених пристроях приведе до зменшення розмірів, підвищення швидкодії та розширення функціональності практичних застосувань. Зазначено, що дослідження, що виконуються в Інституті магнетизму НАН України та МОН України, перебувають у руслі сучасних світових тенденцій. Наведено результати досліджень композитних наноструктур з антиферомагнітним компонентом, а також результати наукової діяльності, спрямованої на розроблення та дослідження магнітних наноматеріалів для медицини, зокрема для самоконтрольованої магнітної гіпертермії.
Цитування:
Товстолиткін О.І. Магнітні нанокомпозити для новітніх технічних та медичних застосувань. Вісник НАН України. 2023. № 2. С. 43—49. https://doi.org/10.15407/visn2023.02.043
Посилання
Coey J.M.D. Magnetism and Magnetic Materials. Cambridge University Press, 2010. https://doi.org/10.1017/CBO9780511845000
Raveendran A., Sebastian M.T., Raman S. Applications of Microwave Materials: A Review. J. Electron. Mater. 2019. 48: 2601—2634. https://doi.org/10.1007/s11664-019-07049-1
Gutfleisch O., Willard M.A., Brück E., Chen C.H., Sankar S.G., Liu J.P. Magnetic Materials and Devices for the 21st Century: Stronger, Lighter, and More Energy Efficient. Advanced Materials. 2011. 23(7): 821—842. https://doi.org/10.1002/adma.201002180
Sai Rama B., Paul A.K., Kulkarn S.V. Soft magnetic materials and their applications in transformers. Journal of Magnetism and Magnetic Materials. 2021. 537: 168210. https://doi.org/10.1016/j.jmmm.2021.168210
Chumak A.V. et al. Advances in Magnetics Roadmap on Spin-Wave Computing. IEEE Transactions on Magnetics. 2022. 58(6): 1—72. https://doi.org/10.1109/TMAG.2022.3149664
Ovcharov R.V., Galkina E.G., Ivanov B.A., Khymyn R.S. Spin Hall Nano-Oscillator Based on an Antiferromagnetic Domain Wall. Phys. Rev. Applied. 2022. 18: 024047. https://doi.org/10.1103/PhysRevApplied.18.024047
Tomasello R., Verba R., Lopez-Dominguez V., Garesci F., Carpentieri M., Di Ventra M., Amiri P.K., Finocchio G. Antiferromagnetic Parametric Resonance Driven by Voltage-Controlled Magnetic Anisotropy. Phys. Rev. Applied. 2022. 17: 034004. https://doi.org/10.1103/PhysRevApplied.17.034004
Afanasiev D., Hortensius J.R., Ivanov B.A., Sasani A., Bousquet E., Blanter Y.M., Mikhaylovskiy R.V., Kimel A.V., Caviglia A.D. Ultrafast control of magnetic interactions via light-driven phonons. Nat. Mater. 2021. 20: 607—611. https://doi.org/10.1038/s41563-021-00922-7
Khymyn R., Lisenkov I., Tiberkevich V., Ivanov B.A., Slavin A. Antiferromagnetic THz-frequency Josephson-like Oscillator Driven by Spin Current. Sci. Rep. 2017. 7: 43705. https://doi.org/10.1038/srep43705
Yu L., Hao L., Meiqiong T., Jiaoqi H., Wei L., Jinying D., Xueping C., Weiling F., Yang Z. The medical application of terahertz technology in non-invasive detection of cells and tissues: opportunities and challenges. RSC Advances. 2019. 17. https://doi.org/10.1039/C8RA10605C
Papaioannou E.Th., Beigang R. THz spintronic emitters: a review on achievements and future challenges. Nanophotonics. 2020. 10(4): 1243—1257. https://doi.org/10.1515/nanoph-2020-0563
Mittleman D.M. Perspective: Terahertz science and technology. Journal of Applied Physics. 2017. 122: 230901. https://doi.org/10.1063/1.5007683
Maxwell L.R., McGuire T.R. Antiferromagnetic Resonance. Rev. Mod. Phys. 1953. 25(1): 279. https://doi.org/10.1103/RevModPhys.25.279
Boventer I., Simensen H.T., Anane A., Kläui M., Brataas A., Lebrun R. Room-Temperature Antiferromagnetic Resonance and Inverse Spin-Hall Voltage in Canted Antiferromagnets. Phys. Rev. Lett. 2021. 126(18): 187201. https://doi.org/10.1103/PhysRevLett.126.187201
Gomonay H.V., Loktev V.M. Spin transfer and current-induced switching in antiferromagnets. Phys. Rev. B. 2010. 81(14): 144427. https://doi.org/10.1103/PhysRevB.81.144427
Polishchuk D.M., Persson M., Kulyk M.M., Holmgren E., Pasquale G., Korenivski V. Tuning thermo-magnetic properties of dilute-ferromagnet multilayers using RKKY interaction. Appl. Phys. Lett. 2020. 117(2): 022402. https://doi.org/10.1063/5.0014823
Ekholm M., Abrikosov I.A. Structural and magnetic ground-state properties of γ-FeMn alloys from ab initio calculations. Phys. Rev. B. 2011. 84(10): 104423. https://doi.org/10.1103/PhysRevB.84.104423
Merodio P., Ghosh A., Lemonias C., Gautier E., Ebels U., Chshiev M., Béa H., Baltz V., Bailey W.E. Penetration depth and absorption mechanisms of spin currents in Ir20Mn80 and Fe50Mn50 polycrystalline films by ferromagnetic resonance and spin pumping. Appl. Phys. Lett. 2014. 104: 032406. https://doi.org/10.1063/1.4862971
Polishchuk D.M., Polek T.I., Kamra A., Kravets A.F., Tovstolytkin A.I., Brataas A., Korenivski V. Spin relaxation in multilayers with synthetic ferrimagnets. Phys. Rev. B. 2018. 98(14): 144401. https://doi.org/10.1103/PhysRevB.98.144401
Polishchuk D.M., Nakonechna O.I., Lytvynenko Ya.M., Kuncser V., Savina Yu.O., Pashchenko V.O., Kravets A.F., Tovstolytkin A.I., Korenivski V. Temperature and thickness dependent magnetostatic properties of [Fe/Py]/FeMn/Py multilayers. Low Temperature Physics. 2021. 47(6): 483. https://doi.org/10.1063/10.0004971
Nogués J., Sort J., Langlais V., Skumryev V., Suriñach S., Muñoz J.S., Baró M.D. Exchange bias in nanostructures. Physics Reports. 2005. 422(3): 65—117. https://doi.org/10.1016/j.physrep.2005.08.004
Polishchuk D.M., Tykhonenko-Polishchuk Yu.O., Lytvynenko Ya.M., Rostas A.M., Gomonay O.V., Korenivski V. Thermal Gating of Magnon Exchange in Magnetic Multilayers with Antiferromagnetic Spacers. Phys. Rev. Lett. 2021. 126(22): 227203. https://doi.org/10.1103/PhysRevLett.126.227203
Polishchuk D.M., Polek T.I., Borynskyi V.Yu., Kravets A.F., Tovstolytkin A.I., Korenivski V. Isotropic FMR frequency enhancement in thin Py/FeMn bilayers under strong magnetic proximity effect. J. Phys. D: Appl. Phys. 2021. 54(30): 305003. https://doi.org/10.1088/1361-6463/abfe39
Duine R.A., Lee K.J., Parkin S.S.P., Stiles M.D. Synthetic antiferromagnetic spintronics. Nature Phys. 2018. 14: 217—219. https://doi.org/10.1038/s41567-018-0050-y
Kravets A.F., Polishchuk D.M., Dzhezherya Yu.I., Tovstolytkin A.I., Golub V.O., Korenivski V. Anisotropic magnetization relaxation in ferromagnetic multilayers with variable interlayer exchange coupling. Phys. Rev. B. 2016. 94(6): 064429. https://doi.org/10.1103/PhysRevB.94.064429
Polishchuk D.M. Ferromagnetic resonance in nanostructures with temperature-controlled interlayer interaction. Low Temperature Physics. 2016. 42(9): 761. https://doi.org/10.1063/1.4964116
Polishchuk D., Tykhonenko-Polishchuk Y., Borynskyi V., Kravets A., Tovstolytkin A., Korenivski V. Magnetic Hysteresis in Nanostructures with Thermally Controlled RKKY Coupling. Nanoscale Research Letters. 2018. 13: 245. https://doi.org/10.1186/s11671-018-2669-0
Polishchuk D.M., Tykhonenko-Polishchuk Yu.O., Holmgren E., Kravets A.F., Tovstolytkin A.I., Korenivski V. Giant magnetocaloric effect driven by indirect exchange in magnetic multilayers. Phys. Rev. Materials. 2018. 2(11): 114402. https://doi.org/10.1103/PhysRevMaterials.2.114402
Borynskyi V.Yu., Polishchuk D.M., Melnyk A.K., Kravets A.F., Tovstolytkin A.I., Korenivski V. Higher-order ferromagnetic resonances in periodic arrays of synthetic-antiferromagnet nanodisks. Appl. Phys. Lett. 2021. 119(19): 192402. https://doi.org/10.1063/5.0068111
Borynskyi V., Kravets A., Polishchuk D., Tovstolytkin A., Sharai I., Korenivski V., Melnyk A. Spin-wave Resonance in Arrays of Nanoscale Synthetic-antiferromagnets. In: IEEE 12th International Conference Nanomaterials: Applications & Properties. Krakow, Poland, 2022. https://doi.org/10.1109/NAP55339.2022.9934337
Périgo E.A., Hemery G., Sandre O., Ortega D., Garaio E., Plazaola F., Teran F.J. Fundamentals and advances in magnetic hyperthermia. Applied Physics Reviews. 2015. 2(4): 041302. https://doi.org/10.1063/1.4935688
Pucci C., Degl'Innocenti A., Gümüş M.B., Ciofani G. Superparamagnetic iron oxide nanoparticles for magnetic hyperthermia: recent advancements, molecular effects, and future directions in the omics era. Biomater. Sci. 2022. 10: 2103—2121. https://doi.org/10.1039/D1BM01963E
Peiravi M., Eslami H., Ansari M., Zare-Zardini H. Magnetic hyperthermia: Potentials and limitations. Journal of the Indian Chemical Society. 2022. 99(1): 100269. https://doi.org/10.1016/j.jics.2021.100269
Yelenich O., Solopan S., Kolodiazhnyi T., Tykhonenko Yu., Tovstolytkin A., Belous A. Magnetic Properties and AC Losses in AFe2O4 (A = Mn, Co, Ni, Zn) Nanoparticles Synthesized from Nonaqueous Solution. Journal of Chemistry. 2015. 2015: 532198. https://doi.org/10.1155/2015/532198
Tovstolytkin A.I. New Functionalities of Nanostructured Oxide Magnetics (by materials of scientific report at NAS Presidium Meeting 15 May 2013). Visn. Nac. Akad. Nauk Ukr. 2013. (6): 7—10. http://doi.org/10.15407/visn2013.06.007
Kalita V.M., Tovstolytkin A.I., Ryabchenko S.M., Yelenich O.V., Solopan S.O., Belous A.G. Mechanisms of AC losses in magnetic fluids based on substituted manganites. Phys. Chem. Chem. Phys. 2015. 17(27): 18087—18097. https://doi.org/10.1039/C5CP02822A
Shlapa Y., Kulyk M., Kalita V., Polek T., Tovstolytkin A., Greneche J.-M., Solopan S., Belous A. Iron-Doped (La, Sr)MnO3 Manganites as Promising Mediators of Self-Controlled Magnetic Nanohyperthermia. Nanoscale Res. Lett. 2016. 11: 24. https://doi.org/10.1186/s11671-015-1223-6
Kalita V.M., Polishchuk D.M., Kovalchuk D.G., Bodnaruk A.V., Solopan S.O., Tovstolytkin A.I., Ryabchenko S.M., Belous A.G. Interplay between superparamagnetic and blocked behavior in an ensemble of lanthanum–strontium manganite nanoparticles. Phys. Chem. Chem. Phys. 2017. 19: 27015—27024. https://doi.org/10.1039/C7CP05547A
Belous A., Tovstolytkin A., Solopan S., Shlapa Yu. Synthesis, Properties and Applications of some Magnetic Oxide Based Nanoparticles and Films. Acta Physica Polonica A. 2018. 133(4): 1006—1012. https://doi.org/10.12693/APhysPolA.133.1006
Solopan S.O., Nedelko N., Lewińska S., Ślawska-Waniewska A., Zamorskyi V.O., Tovstolytkin A.I., Belous A.G. Core/shell architecture as an efficient tool to tune DC magnetic parameters and AC losses in spinel ferrite nanoparticles. Journal of Alloys and Compounds. 88: 1203—1210. https://doi.org/10.1016/j.jallcom.2019.02.276
Tovstolytkin A.I., Lytvynenko Ya.M., Bodnaruk A.V., Bondar O.V., Kalita V.M., Ryabchenko S.M., Shlapa Yu.Yu., Solopan S.O., Belous A.G. Unusual magnetic and calorimetric properties of lanthanum-strontium manganite nanoparticles. Journal of Magnetism and Magnetic Materials. 2020. 498: 166088. https://doi.org/10.1016/j.jmmm.2019.166088
Zamorskyi V.O., Lytvynenko Ya.M., Pogorily A.M., Tovstolytkin A.I., Solopan S.O., Belous A.G. Magnetic Properties of Fe3O4/CoFe2O4 Composite Nanoparticles with Core/Shell Architecture. Ukrainian Journal of Physics. 2020. 65(10): 904. https://doi.org/10.15407/ujpe65.10.904
Nakonechna O.I., Lotey G.S., Kaur J., Bodnaruk A.V., Kalita V.M., Shlapa Yu.Yu., Solopan S.O., Tovstolytkin A.I. AC Field Threshold Effect as a Key Factor toward the Efficient Heating of Fluids with NaFeO2 Magnetic Nanoparticles. Particle & Particle Systems Characterization. 2022. 39(9). https://doi.org/10.1002/ppsc.202200095
Ukrainian physicists will receive international grants. https://www.nas.gov.ua/UA/Messages/news/Pages/View.aspx?MessageID=9553
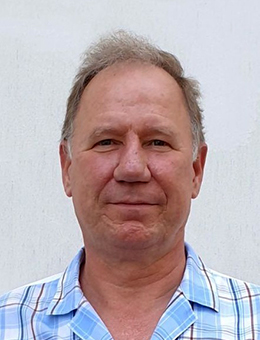